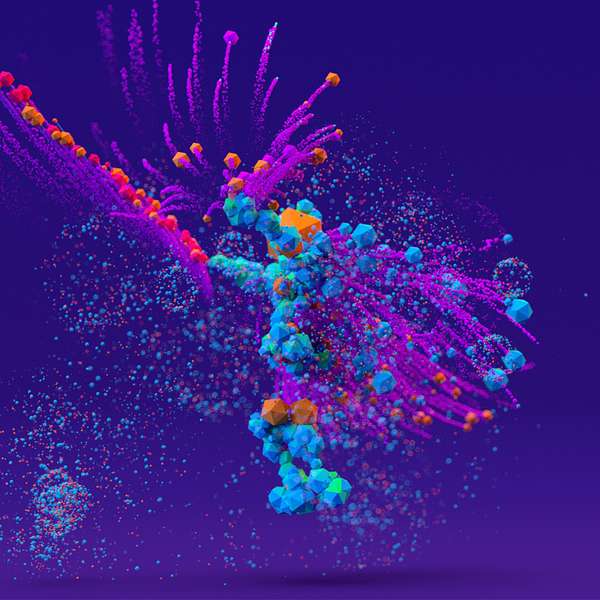
What We Don't Know
What We Don't Know
Quantum gravity
This episode I’ll be exploring one of the greatest mysteries in physics: the incompatibility of general relativity and quantum mechanics. Einstein asserted that gravity is actually the effect caused by space-time curvature. It is very inconvenient that this theory of the very big cannot work with our theory for the very small, the quantum theories that explain how particles behave at the subatomic level.
At the heart of physics nowadays is a quest to create a new theory of quantum gravity, one that explains our universe in the most accurate – and most satisfying – way. First I will talk about some of the science behind each theory, then how they fail together. Finally I’ll dive into some new ideas at the frontier.
https://whatwedontknow.buzzsprout.com/
Hello everyone, welcome to the third episode of ‘What We Don’t Know’, a podcast that explores the boundaries of human knowledge, investigating the unanswered questions and theories that unravel them at the frontiers of science. During this podcast I hope to get you interested in new areas of science, sociology and technology, teaching you about existing concepts and igniting a curiosity for the things we have yet to know.
This episode I’ll be exploring one of the greatest mysteries in physics: the incompatibility of general relativity and quantum mechanics. Einstein, known as one of the greatest physicists ever, developed one of the most important theories of modern physics with his assertion that space and time are part of the same fundamental fabric of the universe, and that gravity is actually the effect caused by space-time curvature. It is very inconvenient that this theory of the very big cannot work with our theory for the very small, the quantum theories that explain how particles behave at the subatomic level. After all, the very big is not independent from the smallest parts that make it up.
At the heart of physics nowadays is a quest to unite these theories, resolve their squabbles, and create a new theory of quantum gravity, one that explains our universe in the most accurate – and most satisfying – way. First I will talk about some of the science behind each theory, then how they fail together. Finally, and most excitingly, I’ll dive into some new ideas at the frontier.
So what actually is general relativity? Newton published his theory of gravity in The Principia in 1687, which described how each particle attracts other particles with a force directly proportional to the product of their masses and inversely proportional to the square of the distance between their centres. Almost 300 years later in 1916, Albert Einstein published a full mathematical framework on general relativity, which reevaluates why bodies demonstrate attraction. In a nutshell, bodies with mass warp space-time: the greater the mass, the greater the curvature.
General relativity’s equations can be shown to predict many astronomical events with a remarkable degree of accuracy, hence its acceptance in the scientific community. For example, general relativity predicts the bending of light around large objects, as well as time dilation, or slowing, closer to the surface of Earth. Both have been proved. Despite the theory’s successes (and it has many) it also has holes, such as the inability to explain singularities inside black holes and the Big Bang. Singularities are locations in space-time where the density and gravitational field of an object are predicted to become infinite, because the warping of space-time is so extreme, but mathematical infinities are usually a sign something has gone wrong, so we’re clearly missing something from our understanding of gravity.
‘Quantum physics’ is more than a throwaway phrase to explain unrelated phenomena in science-fiction movies. It’s rather unintuitive for such an important part of science, even being known for baffling those who understand it the most. Quantum theory was pioneered in large part by a small group of physicists in 1922-1927, physicists including Bohr, Heisenberg, Schrödinger, Dirac and Planck, and it concerns the behaviour of atoms and subatomic particles.
Quantum mechanics – I’ll use quantum physics and quantum mechanics interchangeably, since they’re practically the same thing – says that particles are not really particles, but waves. An electron should be described using a wave function, which is an abstract mathematical wave. By performing mathematical operations on this wave function, we get probability distributions for the electron’s position and velocity. In this way, the electron does not have a concrete position or velocity; instead it is only has probabilities of having certain qualities, where some probabilities may be very high and others very low. It is only when we measure the electron – where measurement is the testing of a physical system to yield a numerical result – that it collapses into a single position and velocity.
In other words, quantum physics says that the universe is inherently probabilistic, that nothing is certain until we measure it. There exists a quantum realm of waves, but we cannot access it, since attempts to observe it give us definite particles. What’s more, nobody knows how the waves actually collapse. This may seem confusing, unintuitive and incorrect, but quantum physics is likely the most successful theory of physics ever, yielding more accurate predictions than even Einstein’s relativity!
Why do general relativity and quantum mechanics not work together? Firstly, quantum mechanics says that an electron is in a superimposed state, being at multiple places at once, producing a position probability cloud around the nucleus. General relativity says mass produces space-time curvature, and electrons have mass, so does the electron warp space-time at every position? Another issue concerns the black hole information paradox, since Hawking radiation implies information loss, but quantum theory forbids destruction of quantum information.
Ultimately, neither theory fully incorporates the other into its predictions. Initially, the Schrödinger equation of quantum physics treated space and time as separate, contradicting Einstein’s special relativity which fundamentally links them. Dirac fixed part of this with a relativistic wave equation, and nowadays quantum field theories fully account for space-time unification, but they do not incorporate the curvature of space-time from general relativity.
Standard quantum theories treat space-time as the sensible stage on which all the weird quantum things happen. It is usual to apply quantum principles to non-gravitational forces of nature: for example, classical electromagnetism becomes quantum electrodynamics. However, a quantised electromagnetic field still lies on a continuous space-time. The gravitational field doesn’t lie on space time – it is space-time – how, then, do we quantise gravity?
The two most famous potential solutions are loop quantum gravity and string theory.
Loop quantum gravity has an interesting name, fit for such an interesting theory. It attempts to quantise the space-time background of reality. In it, space-time is not continuous – as Einstein said – but instead made of discrete quanta. In physics, a quantum is the minimum amount of a physical entity/property involved in a reaction, so this assertion suggests distance has a minimum quantity (as does area, volume, and time). Space-time quanta is made of loops, with nodes at their intersections. The nodes hold quantum volumes, and the loops between nodes are 2D areas. Large quantities of these loops and nodes are called spin networks: space is defined by the geometry of the spin network, and time is defined by its movement. In LQG, time does not flow continuously, but ticks, and each movement of the quantum network is a tick of time. Mass and energy distort the shape and volume of the spin network, which distorts space-time, producing what we perceive as gravity!
Loop quantum gravity is less concerned with the matter that inhabits the universe, and focuses on quantising the fabric of the universe itself. It makes predictions we can test, such as that the speed of light depends on energy, but this effect has not been observed. Predictions like this also go against Einstein’s special relativity, which states the laws of physics should be the same for every observer. One of its biggest problems is that in order for the maths of general relativity to work, space-time has to be smooth at the smallest scales, and LQG does not necessarily ensure that.
String theory is more well known in popular culture. It attempts to provide a completely new mathematical framework for reality, and concerns the matter of the universe rather than the space-time background. In a nutshell, the fundamental particles of the standard model of particle physics are replaced by one-dimensional strings, which can be closed or open; different vibrations of these strings manifest in different behaviours of particles. Vibrating strings producing elementary particles can be thought of like the varying sounds a wind instrument makes depending on the journey of the air.
String theory has its successes. Gravitons, which may carry the gravitational force, emerge naturally from the maths of string theory. Particular sets of strings and vibrations could account for quarks, which make up protons and neutrons. However, in order for modern versions of the theory to work, strings must vibrate in nine spacial dimensions (plus that of time). In the more extreme M-theory, this number goes up to 10. These extra dimensions may be very small and hidden until viewed at minute scales, but we have not yet fully proved their existence. Moreover, string theory requires supersymmetry, the idea that each particle of the standard model has a shadow partner particle with different mass. No trace of supersymmetric particles has been found.
In the quest for a complete theory of quantum gravity, there stands two armies: the loop quantum gravity theorists and the string theorists, who often vehemently disagree. Loop quantum gravity quantises the space-time background, but does not account for other forces, so is not a theory of everything. String theory tries to unite gravity with the standard model of particle physics, but does not produce the space-time background, so is not fully satisfying either.
The quest for a complete theory of quantum gravity is more than abstract maths in conferences and jargon flung around by theoretical physicists. Everything is made up of particles, everything is affected by the universe’s forces; everything exists because of the laws of physics.
Uniting general relativity and quantum mechanics – whether by perfecting the newest theories, combining them, or discovering new concepts we have not yet dreamed about – will develop humanity’s knowledge in magnificent ways. Only then can we reach new heights in our understanding of the true reality of our universe.
Thank you for listening.