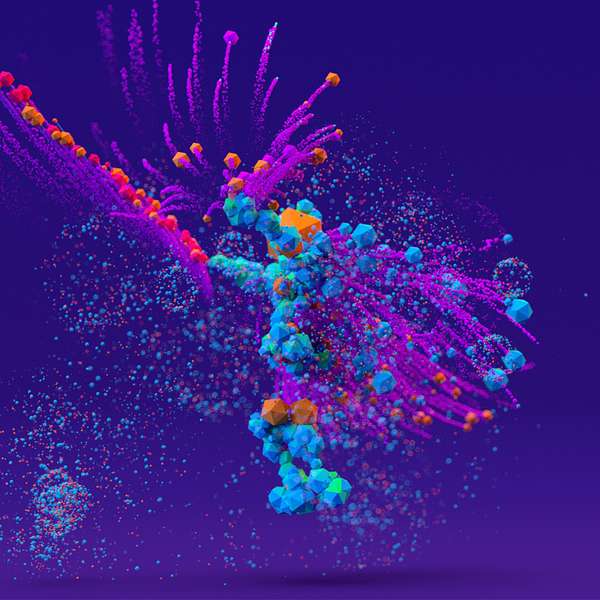
What We Don't Know
What We Don't Know
Silicon-based life
Today we’re talking about the concept of silicon-based life. This is the idea that, instead of carbon being the only building block upon which life has flourished, somewhere in outer space there could be alien life using silicon in its molecular structure.
https://whatwedontknow.buzzsprout.com/
Hello everyone, welcome to the first episode of ‘What We Don’t Know’, a podcast that explores the boundaries of human knowledge, investigating the unanswered questions and theories that unravel them at the frontiers of science. During this podcast I hope to get you interested in new areas of science, sociology and technology, teaching you about existing concepts and igniting a curiosity for the things we have yet to know.
Today we’re talking about the concept of silicon-based life. This is the idea that, instead of carbon being the only building block upon which life has flourished, somewhere in outer space there could be alien life using silicon in its molecular structure.
Before going into silicon as a potential basis for organic life, first we should discuss why carbon is so suitable. A carbon atom has six electrons in total, two of which fill the first shell, leaving four in the outer shell. This number of four makes it incredibly suited to bonding in many different ways. Carbon atoms can form four covalent bonds with other atoms in order to satisfy the octet rule (which states that atoms aim to have a full valence shell of 8 electrons, despite some exceptions), and can do so through single, double or triple bounds. Hydrocarbons – molecules of carbon and hydrogen – can be linear chains, rings, mixtures of both, and the immense geometric variety from different bonding structures gives hydrocarbons a wide range of uses.
To put it simply, carbon atoms can form four covalent bonds, so they can make simple and complex structures relatively easily. Examples include methane and benzene which are hydrocarbons, and glucose, which also contains oxygen. Carbon-carbon bonds are also unusally strong and stable. This bonding potential means carbon forms the backbone of many important organic molecules, such as proteins, DNA, sugars and fats.
What does this mean for alternate life? Science fiction has always dreamt up weird and fantastical alien life: think of E.T and the movie classic Alien; in the original Star Trek there was Horta, the sludgy, silicon-based life form that ate rocks. Here’s where we bring the idea of silicon-based life.
Silicon is directly below carbon in the periodic table, so it also has four electrons in its outer shell. Hypothetically, this should suggest similar suitability to life. Potentially, it could also form complex structures with wide variety, and do so organically on some other plant, or even our own. Moreover, silicon is the 7th most abundant element in the universe. But there are many chemical problems with this theory.
An example of silicon chemistry is the molecule hexasilabenzene, almost the ‘silicon equivalent’ of benzene. Benzene serves as a good example of the flexibility of carbon molecules: it exists as a hexagon ring of carbon with alternative single and double carbon bonds, a hydrogen accompanying each carbon to make the molecule stable. Hexasilabenzene serves as a stark contrast. The structure is disordered, there are two free electrons, and hydrogens are not evenly distributed. Where carbon is stable, silicon is unstable.
Unfortunately, silicon-silicon bonds are much weaker than carbon-carbon bonds. This is shown in hexasilabenzene and makes it more difficult for long chains of silicon to exist stably like carbon chains and rings do. This silicon-bond instability is furthered by silicon’s high reactivity with oxygen. Indeed, a reason there is no silicon-based life on Earth – that we know of – is the high concentration of oxygen in the atmosphere. Most silicon has reacted with oxygen to form silicon dioxide; this is most often recognised in sand and other non-organic minerals. The fact that oxidised silicon is solid, rather than the gas of oxidised carbon, has led to the common comparison of silicon-based life to rocks.
In fact, this revelation may have large consequences on exoplanet gas studies. In most exoplanet gas studies scientists try to find which bio-signature gases are present in the atmospheres of exoplanets and use the data to deduce whether life is likely to exist there. For example, oxygen is a major indicator of life. However, if the exoplanet life would be silicon-based rather than carbon-based, organic life processes such as respiration may involve different gases. Oxygen may even reduce the possibility of silicon-based life. Some say that if silicon-based life breathed in oxygen, the oxygen and silicon would react, and the life form would breathe out solid bricks or dust of silica, which is hydrated silicon dioxide.
One chemical problem difficult to solve is that of handedness. Complex organic carbon molecules can carry out complex processes, and do so with precision, because they have a property called ‘chirality’. This property, whose name comes from the Greek word for ‘handedness’, means that each molecule has a mirror image, the same structurally but unable to fit together. Many carbon life forms take advantage of handedness to form unique structures like glucose and cellulose and to enable enzymes to recognise which reactions to carry out. Unfortunately, silicon cannot form many handed structures that we know of.
Another chemical difference between silicon and carbon compounds are their preferred solvents. Due to the instability of organosilicon bonds, extreme conditions may be needed to sustain them: for example, high temperature and pressure. Because of this, as well as the instability of compounds containing silicon in water, the chances of silicon-based life using water as a solvent are negligible. Despite this, silicon could use different solvents instead, such as ammonia or even low temperature cryosolvents like liquid nitrogen.
In all these ways, silicon-based life seems to need extreme conditions to survive: high temperatures like in the core of the Earth, high pressures to remain stable, or unique combinations of atmospheric gases and solvents to allow silicon to form bonds and carry out organic processes.
Scientists have long known that life on Earth can incorporate silicon into their biochemistry. For example, microscopic particles of silica are found in vascular plants. Photosynthetic algae known as diatoms have cell walls made of silica, called frustules, providing protection and stability.
However, these are only minor incorporations. It is widely agreed that were silicon-based life to exist, it would probably be using silicone, a compound of silicon and carbon. Chemists artificially synthesise silicone regularly, but it is not known for life to produce it naturally. Biology producing silicone seemed a dream; that is, until scientists at Caltech used directed evolution to coax a bacterial protein to make silicon-carbon bonds.
Directed evolution is a method first pioneered in the 1990s by Frances Arnold, where DNA coding for an enzyme is mutated repeatedly, and the new enzymes tested for desirable traits, until the final enzyme works much better than the original. Often this process is used to enhance existing enzymes, but Arnold and her lab did new research to persuade an enzyme to do something new.
They used a protein called cytochrome c from a bacterium living in Icelandic hot springs. The protein ordinarily shuffles electrons to other proteins, but the researchers found it can act as an enzyme to catalyse silicon-carbon bond formation at low levels. After three rounds of DNA mutations in the region thought to be responsible for the portion of protein responsible for organosilicon bond production, the lab had created an enzyme that could selectively make silicon-carbon bonds 15 times more efficiently than the best catalysts created by chemists. They described the genetically encoded enzyme as producing fewer unwanted by-products, non-toxic, cheaper, and easier to modify than inorganic alternatives.
Aside from the industrial benefits of a more effective silicon-carbon bond producer, the research clearly shows the potential of life to form organosilicon bonds. If scientists in a lab can force proteins to do so, there should be no reason nature would refuse.
In this episode we discussed why carbon was suited for life and its implications for silicon, the chemical differences between carbon and silicon compounds that posed large problems, and the potential solutions to these obstacles. Finally, we touched on some existing instances of silicon in biology, and the power of bioengineering and DNA editing to make life that produced silicone naturally.
Silicon-based life may seem unlikely. The conditions required may forbid complex organic structures, so if silicon-based life does exist out there, it may be very basic. However, we should not let an Earth-centric view restrict our theorising. Silicon-based life could expel solid waste products through obscure means, such as shedding shells. Furthermore, there is currently no proof that exoplanets with the perfect conditions for silicon-based life, do not exist. Organic processes could work around the problem of handedness.
When considering silicon-based life, we often think of basic rock structures, similar to the primarily-silicon crust of our Earth. But by that logic, maybe carbon-based life should just be graphite or diamonds? We must keep our minds open to all possibilities of alternative life, no matter how bizarre, and remember the extraordinary complexity nature often devises, even in the harshest and most improbable of conditions.
Silicon is a hugely useful element, used to make many inorganic materials, used in alloys, computer chips, solar cells and semi-conductors. Maybe one day we’ll utilise it to make life as well. Maybe silicon-based life already exists, somewhere in the vastness of space.
Thank you for listening.